The Fuel Cell Technologies Office's (FCTO's) chemical hydrogen storage materials research focuses on improving the volumetric and gravimetric capacity, transient performance, and efficient, cost-effective regeneration of the spent storage material.
The Hydrogen Storage Engineering Center of Excellence has developed a system projection graph showing a modeled ammonia borane system and how it compares against all of DOE's 2020 targets.
Download the final report for the DOE Chemical Hydrogen Storage Center of Excellence.
Technical Overview
The category of chemical hydrogen storage materials generally refers to covalently bound hydrogen in either solid or liquid form and consists of compounds that generally have the highest density of hydrogen. Hydrogen release from chemical hydrogen systems is usually exothermic or has a small endothermic enthalpy; thus, rehydrogenation typically requires the dehydrogenated product to be rehydrogenated off-board by processes other than through the application of hydrogen pressure. This approach can be used for single-use, high-value-added applications, but consideration of chemical hydrogen storage for large-scale transportation use will require off-board rehydrogenation of the spent product, which at present greatly increases the well-to-power-plant fuel cost. Moreover, as with complex hydrogen compounds, release from chemical hydrogen compounds can have reaction pathways that may not be easily predicted.
The dehydrogenation of chemical hydrogen itself can be accomplished either hydrolytically by reaction with water1 or thermolytically by heating the compound, as with ammonia borane (NH3BH3)2 and alane (AlH3).3 Given their high hydrogen capacity, ammonia borane and amide and amine compounds and variants remain systems of interest. As in the case of complex hydrides, the reaction pathway for dehydrogenation of chemical hydrogen is not necessarily straightforward and may be governed to the extent to which the dehydrogenation product remains as a liquid phase or slurry that can be handled easily.
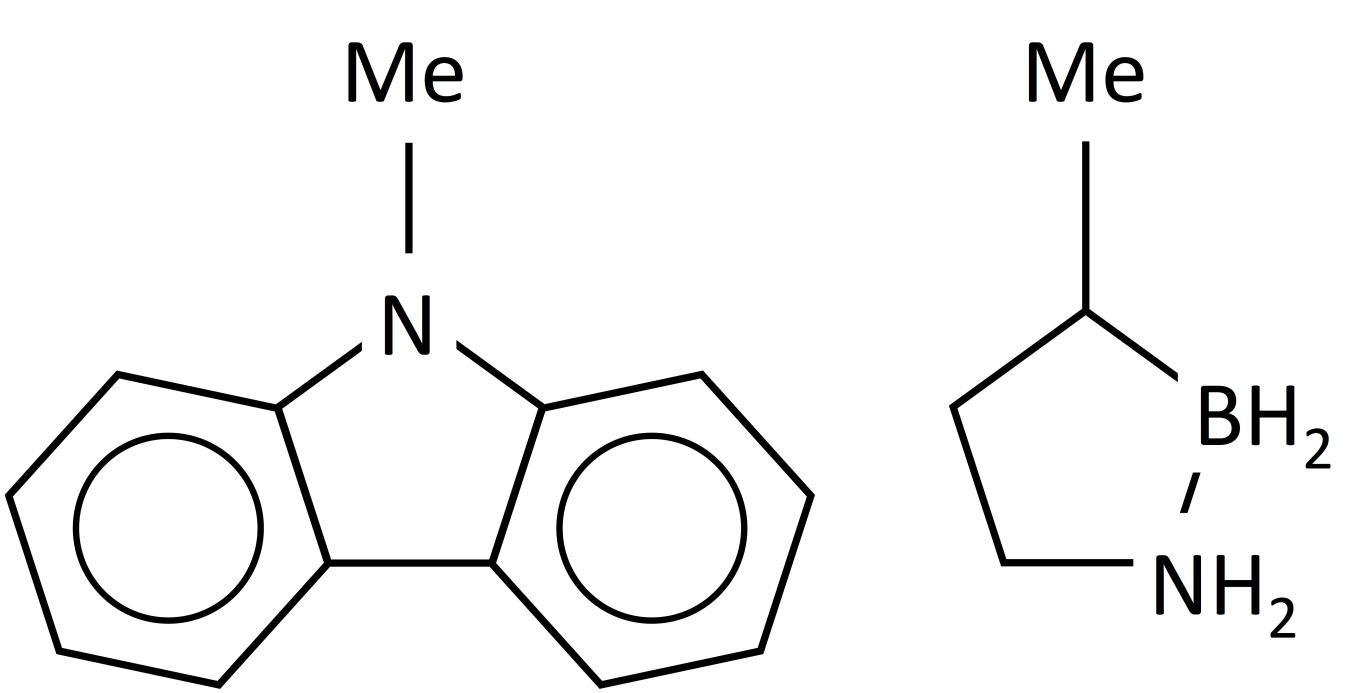
Structures of n-ethylcarbazole (left) and methyl cyclopentane (right).
In addition to covalently bound hydrogen as solids, compounds that are capable of binding hydrogen as liquids have been studied. Examples of systems based on liquid carriers include n-ethylcarbazole4 and methyl-cyclopentane5 as shown in the figure. In addition to the need for off-board rehydrogenation of the spent product, some of the difficulty in working with these liquids is that the dehydrogenated product can solidify, complicating the handling of the spent materials, thus affecting their overall utility.
References
- Kojima, Y.; Kawai, Y.; Kimbara, M.; Nakanishi, H.; Matsumoto, S. "Hydrogen Generation by Hydrolysis Reaction of Lithium Borohydride." International Journal of Hydrogen Energy (29), 2004; pp. 1213–1217.
- Gutowska, A.; Li, L.; Shin, Y.; Wang, C.M.; Li, X.S.; Linehan, J.C.; Smith, R.S.; Kay, B.D.; Schmid, B.; Shaw, W.; Gutowski, M.; Autrey, T. "Nanoscaffold mediates hydrogen release and the reactivity of ammonia borane." Angewandte Chemie International Edition (44), 2005; pp. 3578–3582.
- Graetz J.; Reilly, J.J. "Kinetically stabilized hydrogen storage materials." Scripta Materialia (56:10), 2007; pp. 835–839.
- Cooper, A.C.; Fowler, D.E.; Scott, A.R.; et al. "Hydrogen storage and delivery by reversible hydrogenation of liquid-phase hydrogen carriers." Abstracts of Papers of the American Chemical Society (229), 2005; pp. U868–U868, Part:1 Meeting Abstract: 113-FUEL.
- Luo, W.; Campbell, P.G.; Zakharov, L.N.; Liu, S.Y. "A Single-Component Liquid-Phase Hydrogen Storage Material." Journal of the American Chemical Society (135:23), 2013; pp. 8760–8760.